Head of the Laboratory: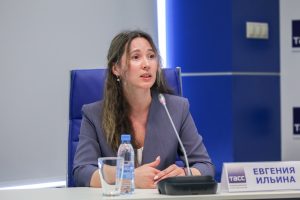
Evgeniya Il’ina
PhD (Chemistry)
E-mail:ilyina[at]ihte.ru
WebPage
The Laboratory was found in 1962 by Gennady Stepanov, PhD (Chemistry) and called the Fuel Cells Laboratory. In 1980 the name was changed to the Laboratory of Electrochemical Power Sources. Nikolay Batalov, PhD (Chemistry) and Honorary Scientist of Russian Federation headed the laboratory for the following 30 years (1985-2015).
There are 22 research fellows, including 2 DSc (Chemistry), 8 PhD (Chemistry).
Web pages of the research fellows
_____________________________________________________________________________________________________
The research performed in the laboratory is supported by grants of the Russian Science Foundation. R&Ds are performed with the following industrial partners: Nissan, LG electronics, Samsung SDI, JSC Uralelement, JSC “Irkutsk Electric Grid Company” and other.
Among the main achievements:
-
A new method for synthesis of metal-graphene composites by the interaction of carbon-containing precursors with molten aluminum or its alloys with copper, manganese, magnesium, silicon, or iron under a layer of molten alkali metal halides. The carbon formed inside the metal matrix is a defect-free 2-3 layer graphene; its films are evenly distributed inside the aluminum matrix; the electrical and thermal conductivities are increased by 10-15%. At 300 C, aluminum-graphene composites exhibit abnormally high ductility.
-
The discharge characteristics of elements of a thermally activated chemical current source (TCSS) containing mixtures of transition metal halides and oxides as positive electrodes. Methods for active components and separators production for the studied TCSS have been worked out. Structural solutions for the high power TCSS batteries design, capable of operating in a wide range of climatic conditions and adapted to significant mechanical loads are presented. Recent developments make it possible to ensure the battery cell operation up to 1 hour with the ability to operate in a wide range of ambient temperatures (from -60 to 60°C).
-
A fireproof aluminum-ion battery (AIB) capable of operating at room and negative temperatures. The density, viscosity, electrical conductivity, and ion transfer numbers of chloraluminate ionic liquids (ILs) have been systematically studied in a wide range of aluminum trichloride content at temperatures from -30 to 100°C. Based on the fundamental research on the properties of ILs and the mechanisms of electrode reactions, an AIB prototype with an aluminum-graphene anode, a carbon cathode, and an acidic IL (AlCl3–1-ethyl-3-methylimidazolium chloride) were developed. Distinctive features of AIB are fast charging, high Coulomb efficiency and retention of the capacity of the cathode material for 3000 charge/discharge cycles in the galvanostatic mode.
-
Optimization of the composition and conductivity data, as well as methods for the synthesis of glassy, ceramic, composite, and glass-ceramic electrolytes with lithium, sodium, and potassium cation conductivity. The most highly conductive compositions have values of unipolar lithium-ion conductivity of ~10–3–10–4 S/cm at room temperature. For a number of lithium and sodium phases, which are of interest as electrode materials and solid electrolytes for all-solid-phase chemical current sources, the relationship between transport properties and details of the crystal structure in a wide temperature range have been studied. Layered oxide nanopowders were obtained in the M2O – V2O5 (M=Li, Na, K) system, which are able to easily cover the surface of a solid electrolyte, providing a tight contact between two solid phases in the all-solid-phase current sources. The chemical stability of solid electrolytes with lithium- and sodium-cation conductivity with respect to the corresponding alkali metal both in the currentless mode and during charge-discharge cycling, as well as the stability of these solid electrolytes in contact with electrode materials based on vanadium bronzes at room and elevated temperatures. A strategy is proposed for organizing a tight contact at the solid electrolyte–electrode interface by introducing a lithium-conducting glassy or low-melting additive into LiCoO2 and Li4Ti5O12. In order to reduce the resistance at the interface between the Li anode and the solid electrolyte, it is proposed to introduce 150 nm of Al or switch to the Li-In alloy formed by the in-situ method.
-
In the field of carbonate fuel cell technology (CFC), extensive studies have been carried out on the physicochemical properties of carbonate melts, thermodynamics of equilibria in fuel and oxidizing gas mixtures, and the electrode processes. Successful bench tests of 200 W CFC stacks with external conversion of natural gas were carried out for 2000 hours. Since 2015, studies have been underway on the applicability of the CFC technology to capture carbon dioxide from air and industrial gases. The installation for carbon dioxide capturing from the air in closed rooms, that are based on CFC, were tested in collaboration with the Keldysh Center (Moscow). The high efficiency of the technology was shown even at such low concentrations of CO2 as 0.5 – 2%.